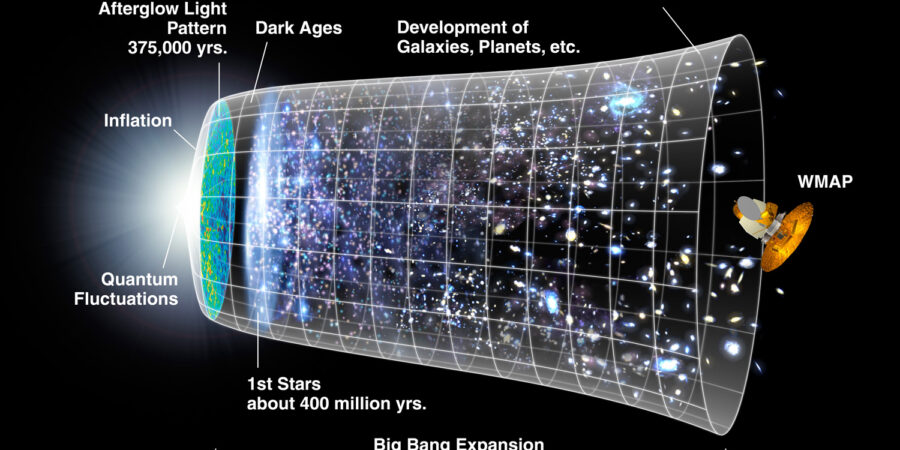
Cosmology is the study of the universe as a whole. We try to understand how it came into being, how it is currently growing and how it will eventually die. Over the last few decades, cosmologists have developed the so-called “Lambda CDM” standard model of cosmology, which explains the vast majority of current large-scale observations. However, this model relies on two unknown quantities: the “Lambda”, which refers to the cosmological constant, and the “CDM”, for cold dark matter. The cosmological constant is one option to explain why the expansion of the Universe is currently accelerating; other solutions would be to introduce a dark energy component or to modify the laws of gravity. Dark matter is invoked to explain gravitational interactions that are not associated with luminous matter, and could also be explained by modified gravity models. Together, the cosmological constant and dark matter account for about 95% of the energy budget of the Universe, which means that only 5% of the Universe is visible. At the Laboratoire d’Astrophysique de Marseille, we are trying to observe this dark Universe with different techniques to improve our understanding of the evolution of the Universe and to test all these alternatives to the standard model of cosmology.
-
Cosmic Microwave Background
The Cosmic Microwave Background (or CMB) is the first light that was emitted by the Universe. As such, it contains information about the Universe when it was very young.
Our understanding of the cosmos is punctuated by a few rare essential observations. By producing the “ultimate” map of the CMB intensity, the Planck satellite definitively contributed to the history. The information extracted from Planck gives a great confirmation of the standard model of cosmology with an unprecedented accuracy. However, because Planck precision is so high, it also made it possible to reveal some unexplained features that could require new physics to be understood. Future CMB experiments will help to better understand these features but they will especially aim at going back even further in time, closer to the Big Bang.
Such kind of millimeter-wave experiments also contains information about the large-scale structure in the CMB foreground, as the CMB photons passed through the entire Universe and interacted with many objects before reaching us.
In particular, the Cosmic Infrared Background (CIB) fluctuations are one of these foregrounds. They are produced by the collective unresolved emission of distant galaxies. In our team, we are modeling the CIB such as to extract the cosmological information from CMB data, and we use the CIB to measure the history of star formation and to study the relation between star formation and the cosmic web.
-
Large-scale structure
Large-scale structure forms in the late Universe, when it becomes cold enough for matter to accrete and organize itself on what we call the cosmic web. Galaxies are found in large filaments of matter that are connected by clusters of galaxies, another important subject studied in our laboratory. These filaments are surrounded by sub-dense volumes that we call voids. This large-scale structure traces both the expansion history of the Universe and its matter distribution, and is thus an efficient cosmological probe.
To extract this information, we study matter clustering, the way galaxies are distributed in the sky, and gravitational lensing, the deflection of light due to massive objects. Many researchers from the Laboratoire d’Astrophysique de Marseille are involved in the future instruments dedicated to these measurements: the Euclid satellite, the Roman satellite and the Rubin Observatory. Within these large international collaborations, we are developing new statistical tools to exploit this wealth of future data: correlation functions, higher order statistics, machine learning… In particular, we are very excited about the upcoming launch of the Euclid satellite which will observe one third of the sky in order to understand through these large scale structure analyses what is driving the accelerated expansion of the Universe.
-
High energy emissions from dark matter
Galaxy cluster haloes are massive storage ring of dark matter and represent interesting target for dark matter indirect detection. We study the possible emissions from dark matter annihilation or decay in nearby galaxy clusters to derive constraints on dark matter particle candidate properties and on dark matter distribution in clusters. Those studies are relevant for gamma-rays astronomy with experiments like FERMI, HESS/CTA as well as neutrino telescopes like IceCube, KM3NeT.